By OncoPept Team: Papia Chakraborty, Vasumathi Kode, Coral Karunakaran, Kayla Lee, Tahira Khan, Keshav Bhojak, Athulya Ramesh, Sudheendra HV, Rekha Sathian, Ankita Srivastava, Malini Manoharan, Swapnil Mahajan, Amitabha Chaudhuri
Race for Developing COVID-19 Vaccines
COVID-19 pandemic has infected over 23 million individuals and claimed over 800,000 lives globally as of August 2020. SARS-CoV-2, the organism causing COVID-19 belongs to the family of coronaviruses and shares 79% genome sequence identity with SARS-CoV. The spike antigen used by the virus to enter host cells became the prime target for immediate vaccine development efforts because of prior work on SARS-CoV that showed neutralizing antibodies against the spike antigen protected mice and chimps against new infection (1, 2). Currently, over 166 vaccines are in development against SARS-CoV-2, which can be divided into two major categories – nucleic acid (DNA/RNA) and protein-based (antibodies, viral proteins, inactivated/attenuated viruses and virus-like particles (3, 4) vaccines. Four vaccine candidates – RNA-based delivery of spike protein (Moderna), RNA-based delivery of receptor-binding domain (RBD) of spike protein (Pfizer/Biontech), DNA-based delivery of spike protein (Inovio) non-replicating adenovirus-based delivery of spike protein (Oxford/AstraZeneca and CanSino Biologics) and inactivated virus (Sinovac) are in Phase-I or have completed Phase-I/II trials (5-9). Preliminary data from some of these trials show the induction of neutralizing antibodies against the spike antigen. T-cell immunity is relatively less robust in treated individuals. At present, efficacy data are lacking to support whether the induction of neutralizing antibodies alone will be sufficient to protect individuals from new infections.
Several features of SARS-CoV-2 infection are unique and do not follow the path of other respiratory viruses. For example, infected individuals remain asymptomatic carrying high viral load thereby becoming a potent source of viral transmission. Further, the immune response against SARS-CoV-2 is skewed towards a TH1/TH2 CD4 T-cell response, which results in severe immune toxicity in 15-20% of infected individuals (12, 13). Based on studies in other respiratory viruses, the development of protective immunity against SARS-CoV-2 in vaccinated individuals may face certain challenges. First, the mucosal antibody response is short-lived against respiratory viruses and shows a similar trend in SARS-CoV-2 raising the concern about whether an antibody response is sufficient for long-term protection (10). Second, some vaccinated individuals can experience life-threatening immune toxicity when exposed to the virus (11). Our understanding of how the immune system interacts with the vaccine and how this interaction will translate to a response during active infection remains rudimentary and therefore a source of concern especially in older individuals who are both susceptible to infection and also to immune toxicity, but need the vaccine most urgently. A good vaccine will engage both T-cell and B-cell immunity to provide immediate pathogen clearance and induce memory for long-term protection.
B and T-Cells in Adaptive Immunity
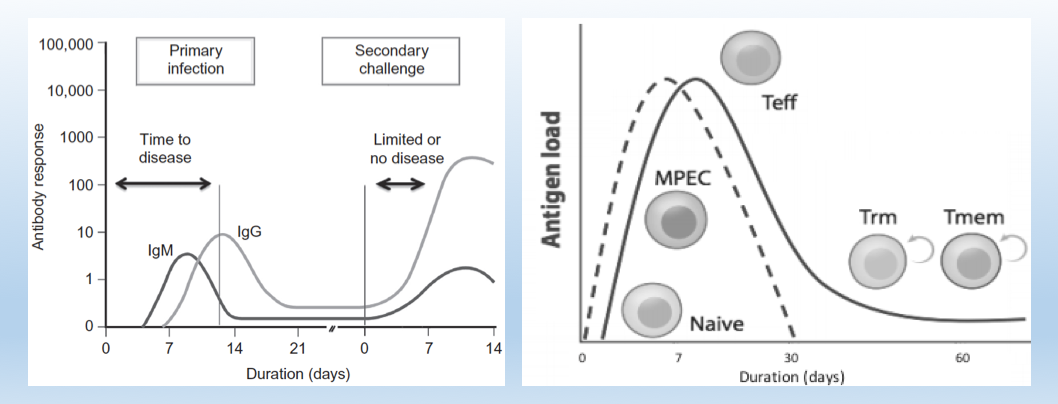
Image sources: Introductory Immunology, 2nd Edition, Modified from CD8 T Cell Exhaustion During Chronic Viral Infection and Cancer, Annual Reviews of Immunology 2019.
Historically, the B-cell memory arm responsible for pathogen-specific neutralizing antibodies is characterized in greater detail in vaccine development studies. Figure 1 (left) demonstrates how B-cells secreting IgM molecules provide short-term clearance of pathogens and pathogen-infected cells, while concurrently maturing into IgG secreting plasma cells that confer long-term protection. The kinetics of the T-cell arm Figure 1 (right) follows a similar profile where antigen-experienced T-cells expand in number, differentiates into a killer phenotype, and clears infected cells. Following clearance of the infection, T-cells persist as resident memory cells in the tissues and as effector memory cells in circulation becoming sentinels to prevent future infections (1). While the B-cell arm of adaptive immunity has been widely emphasized in vaccine development, the T-cell mediated immunity has remained underexplored primarily due to lack of the right technologies. However, significant technological advancements in the last decade have galvanized the study of T-cell mediated immunity in human diseases.
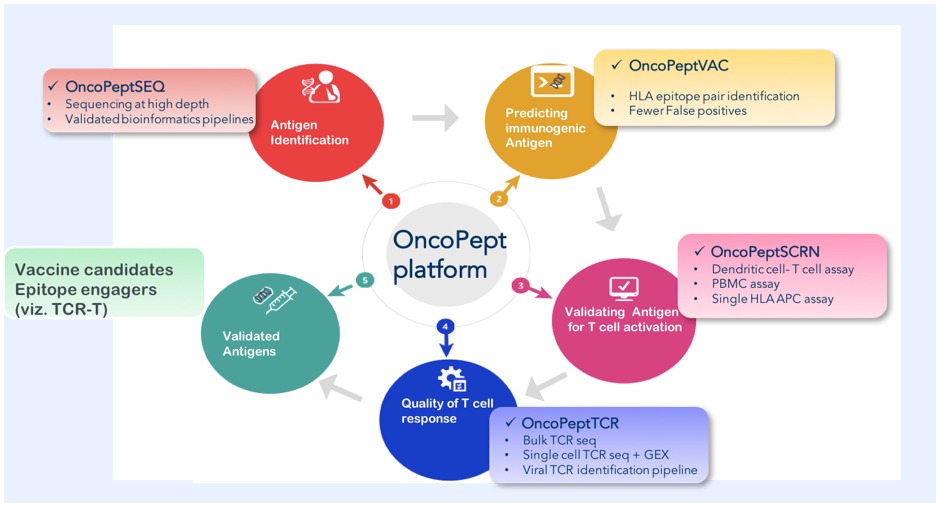
The activation of T-cells is MHC-peptide dependent. T-cell receptors (TCR) on CD8+ cytotoxic T-cells and CD4+ helper T-cells bind 9-15-mer peptide fragments in complex with MHC (referred to as HLA for humans). These peptides are recognized as foreign (non-self) and activate T-cells to generate protective immunity. A large diversity of MHC genes and their polymorphic variants bind millions of peptide fragments from proteins and non-protein antigens and present them to a large diversity of T-cells expressing ~ 106 – 109 unique TCRs. A single peptide can mobilize and activate many T-cells each expressing a unique TCR. Identifying a good TCR-MHC-peptide pair that can clear pathogens is the holy grail of cellular immunology. Figure 2 is a schematic of MedGenome’s OncoPept platform that identifies potent MHC-peptide-TCR combinations for efficient pathogen clearance and protective immunity.
T-Cells and COVID-19
In early April, the OncoPept team conducted their very first immune assays with peptide pools from the spike antigen of SARS-CoV 2 and were surprised to observe pre-existing T-cell immunity in healthy donors who were unexposed to the virus. Sequence alignment analysis revealed the possibility that a part of the global population may be protected against the current pandemic as a result of pre-existing immunity against other ‘’common cold viruses’’ in the Corona virus family. In fact a recent study in Cell reports that many healthy individuals have both CD4+ and CD8+-T cells that elicit an antigenic response to the new coronavirus even though they have never been exposed to SARS-CoV or MERS (2).
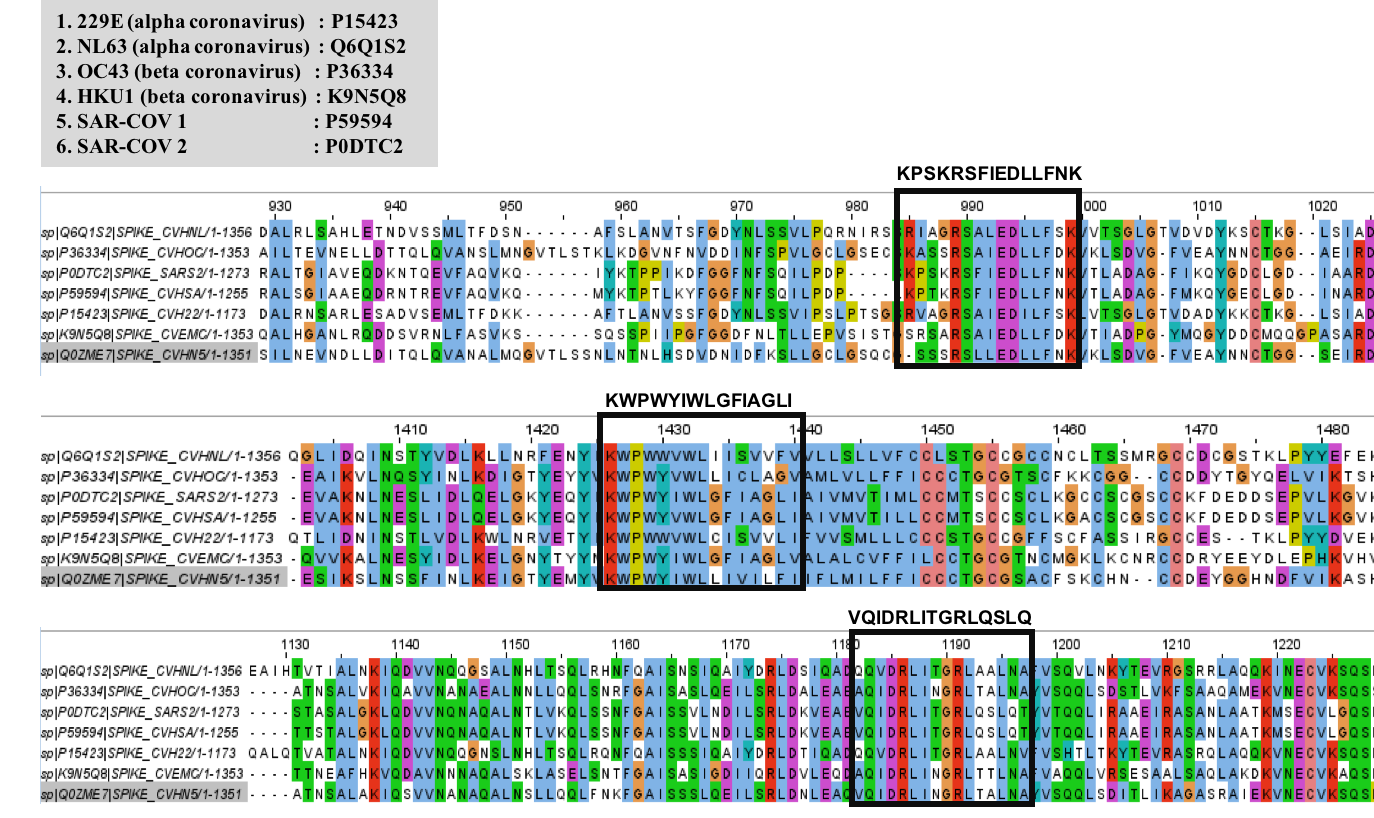
Going back to clues on de novo CD8 immunity against SARS-CoV-2, earlier infection by SARS-CoV can perhaps shed some key insights since it is one of the closest homologs in the phylogenetic tree. Patients who had recovered from SARS-CoV back in 2003 had indeed presented an antibody response that faded within two or three years. Interestingly these patients also elicited detectable virus-specific robust T cell response 10-17 years after the infection had disappeared (3, 4). These startling pieces of evidence support the notion that perhaps targeting T-cell driven immunity may be a key to a robust long-term immune protection against the COVID-19 pandemic in the population (5).
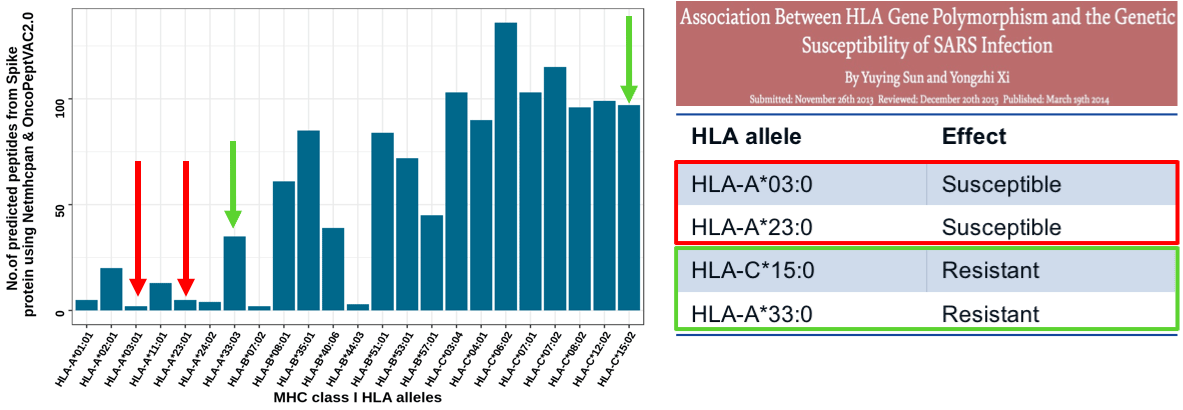
The OncoPept platform was used to assess CD8 T-cell-targeted immunity to the spike protein demonstrated to be highly immunogenic in SARS-CoV studies (6). The algorithm identified CD8 T-cell epitopes restricted to 23 HLAs. Figure 4 shows certain HLAs were restricted to a small number of peptides, whereas other HLAs presented many peptides. If an HLA presents a few peptides from a pathogenic organism the immune system may not register its presence and will fail to mount a protective response. Our prediction analysis indicated that individuals harboring HLA-A:03 or A:23 will present fewer peptides for immune recognition and individuals carrying these HLAs may be more susceptible to infection. Interestingly, a study showed that individuals carrying HLA-A*03 and A*23 were indeed more susceptible to SARS-CoV than individuals carrying HLA-C*15 and HLA-A*33, which bound many more peptides (Figure 4). The data is purely correlational but supports the idea that CD8 T-cell immunity is critical for developing a robust protective SARS-CoV-2-specific immune response.
Possible Challenges in Designing COVID-19 Vaccines
Published studies from Phase-I/II vaccine clinical trials by different groups have indicated a milder CD8 T-cell response against the spike antigen (8, 9). It is possible that potent T-cell response may lie in regions outside the spike protein as shown by the presence of memory T-cell responses against nucleocapsid protein and the non-structural proteins NSP7 and NSP13 gene products from the ORF-1 region in convalescent individuals (4). Identification and characterization of these epitopes and their inclusion in a selected vaccine cocktail may yield a more robust and targeted T-cell protective effect.
Another important consideration while using full-length Spike antigen over “selected epitopes” with immunogenic potential is the phenomenon of epitope dominance. The use of a full-length antigen may result in immuno-dominant T-cell responses outcompeting sub-dominant ones. In many scenarios, these immuno-dominant epitopes saturate MHC molecules on cells and mobilize large families of TCRs with reduced cytotoxic potential. This is a common mechanism employed by viruses as a means of evading the immune system. The response can be made broader and potent by utilizing a rational selection of sub-dominant T-cell epitopes or immuno-dominant epitopes that mobilize a productive and robust cytotoxic response for a well-rounded T-cell immunity (13, 14)
An important hallmark of SARS-CoV-2 infection is viral pneumonia accompanied by pulmonary inflammation and edema characterized by eosinophilic infiltrates. A current study highlights the critical role of host TH17 inflammatory responses in mediating this process. Elevated TH17 responses were also observed previously in MERS-CoV and SARS-CoV patients. Also, studies in experimental animals using vectored vaccines have reported substantial immune enhancement in both the lungs and liver of experimental animals characterized by eosinophilic infiltrates (15, 16). Therefore, careful parsing of T-cell epitopes is much needed to prevent the presentation of TH17-inducing epitope elements increasing the chances of immune toxicity and disease susceptibility.
Conclusion
To conclude, a wide array of respiratory viruses induces severe pneumonia, bronchitis, and even death following infection. Despite this immense clinical burden, there is a lack of efficacious vaccines with long-term therapeutic benefit. Most current vaccination strategies employ the generation of broadly neutralizing antibodies, however, the mucosal antibody response to many respiratory viruses is short-lived and declines with age. In contrast, several studies on respiratory viruses have shown the presence of robust virus-specific CD8-T cell responses which has been shown to last for decades. Therefore, vaccine designs for emerging respiratory viruses need consideration and rational inclusion of CD8 epitopes to confer long term resistance. The OncoPept platform developed at MedGenome combines computational and experimental methods to mine strong CD8 immunomodulatory antigens with therapeutic utility across disease areas spanning respiratory and other infectious diseases, cancer, and autoimmunity.
References
- Actor JK. Introductory Immunology: Basic Concepts for Interdisciplinary Applications. 1st ed2014 June 2014.
- Grifoni A, Weiskopf D, Ramirez SI, Mateus J, Dan JM, Moderbacher CR, et al. Targets of T Cell Responses to SARS-CoV-2 Coronavirus in Humans with COVID-19 Disease and Unexposed Individuals. Cell. 2020;181(7):1489-501 e15.
- Ng OW, Chia A, Tan AT, Jadi RS, Leong HN, Bertoletti A, et al. Memory T cell responses targeting the SARS coronavirus persist up to 11 years post-infection. Vaccine. 2016;34(17):2008-14.
- Le Bert N, Tan AT, Kunasegaran K, Tham CYL, Hafezi M, Chia A, et al. SARS-CoV-2-specific T cell immunity in cases of COVID-19 and SARS, and uninfected controls. Nature. 2020;584(7821):457-62.
- Schmidt ME, Varga SM. The CD8 T Cell Response to Respiratory Virus Infections. Front Immunol. 2018;9:678.
- Subbarao K. SARS-CoV-2: A New Song Recalls an Old Melody. Cell Host Microbe. 2020;27(5):692-4.
- Jackson LA, Anderson EJ, Rouphael NG, Roberts PC, Makhene M, Coler RN, et al. An mRNA Vaccine against SARS-CoV-2 – Preliminary Report. N Engl J Med. 2020.
- Folegatti PM, Ewer KJ, Aley PK, Angus B, Becker S, Belij-Rammerstorfer S, et al. Safety and immunogenicity of the ChAdOx1 nCoV-19 vaccine against SARS-CoV-2: a preliminary report of a phase 1/2, single-blind, randomised controlled trial. Lancet. 2020;396(10249):467-78.
- Mulligan MJ, Lyke KE, Kitchin N, Absalon J, Gurtman A, Lockhart S, et al. Phase 1/2 study of COVID-19 RNA vaccine BNT162b1 in adults. Nature. 2020.
- Walsh EE, Frenck R, Falsey AR, Kitchin N, Absalon J, Gurtman A, et al. RNA-Based COVID-19 Vaccine BNT162b2 Selected for a Pivotal Efficacy Study. medRxiv. 2020.
- Long QX, Tang XJ, Shi QL, Li Q, Deng HJ, Yuan J, et al. Clinical and immunological assessment of asymptomatic SARS-CoV-2 infections. Nat Med. 2020;26(8):1200-4.
- Scherer A, Salathe M, Bonhoeffer S. High epitope expression levels increase competition between T cells. PLoS Comput Biol. 2006;2(8):e109.
- Im EJ, Hong JP, Roshorm Y, Bridgeman A, Letourneau S, Liljestrom P, et al. Protective efficacy of serially up-ranked subdominant CD8+ T cell epitopes against virus challenges. PLoS Pathog. 2011;7(5):e1002041.
- Ruckwardt TJ, Luongo C, Malloy AM, Liu J, Chen M, Collins PL, et al. Responses against a subdominant CD8+ T cell epitope protect against immunopathology caused by a dominant epitope. J Immunol. 2010;185(8):4673-80.
- Hotez PJ, Bottazzi ME, Corry DB. The potential role of Th17 immune responses in coronavirus immunopathology and vaccine-induced immune enhancement. Microbes Infect. 2020;22(4-5):165-7.
- Wu D, Yang XO. TH17 responses in cytokine storm of COVID-19: An emerging target of JAK2 inhibitor Fedratinib. J Microbiol Immunol Infect. 2020;53(3):368-70.
#COVID-19 Vaccines, #Adaptive Immunity, #B-cell memory, #T-cell, #Epitopes, #HLA